Description #
This unit is designed to help the students and clinically registered nurses develop a better understanding of the skills involved in therapeutic oxygen administration.
Learning Objectives #
Upon completion of this unit you will be able to:
1. Describe the principles of oxygenation.
2. Understand the function and limitations of pulse oximetry.
3. Identify indications for oxygen therapy.
4. Describe the capabilities and limitations between low flow and high flow oxygen therapy systems.
5. Follow an algorithm for applying and titrating oxygen therapy.
6. List precautions and complications of oxygen therapy.
Introduction
OXYGEN THERAPY
Oxygen is a common part of patient care in both acutehospital and community settings. This unit is designed to help you develop a better understanding of the theory and skills involved in therapeutic oxygen administration for patient care.
USEFUL DEFINITIONS:
FiO2: Fractional Inspired Oxygen. FiO2 is the oxygen percentage written as a decimal.
Room air contains 21% oxygen or an FiO2 of 0.21. Oxygen from a flowmeter has an FiO2 of 1.00.
PaO2: Partial Pressure of Oxygen in arterial blood. An indirect measurement of the amount of oxygen dissolved in the plasma.
PaCO2: Partial Pressure of Carbon Dioxide in arterial blood. An indirect measurement of the amount of carbon dioxide dissolved in the plasma.
SpO2: O2 Saturation measured by Pulse Oximetry. A noninvasive measurement of the amount of oxygenated hemoglobin. An SpO2 of 95% indicates that 95% of the hemoglobin molecules are carrying oxygen.
SaO2: O2 Saturation measured by Cooximeter. A direct measurement of the amount of oxygenated hemoglobin in an arterial blood sample. Can also measure dysfunctional hemoglobin such as carboxyhemoglobin and methemoglobin.
Principles of oxygenation #
1. Describe the principles of oxygenation
The normal PaO2 of the healthy adult is 80-100 mmHg. Only about 3% of the oxygen carried in the blood is dissolved in the plasma (this portion can be assessed by looking at the PaO2). The vast majority of oxygen carried in the blood is attached to hemoglobin (this portion can be assessed by looking at the SpO2 or SaO2). Therefore, the saturation values are more clinically significant than the PaO2 in determining the oxygen content of the blood. The hemoglobin molecule contains four binding sites for O2. Under normal conditions, all four sites are filled to capacity (fully saturated).
The hemoglobin holds oxygen in reserve until such time that the metabolic demands of the body require more O2. The Hgb then feeds the oxygen to the plasma for transport to the tissues.
The body’s demand for oxygen is affected by:
- Activity
- Metabolic status
- Temperature
- Anxiety
The ability of Hgb to feed the oxygen to the tissues depends on a number of factors such as:
- O2 supply
- Ventilatory effectiveness
- Nutrition
- Cardiac output
- Hgb content
- Smoking
- Drugs
- Diseas
Any one of these factors can impede the supply and transport of oxygen to the tissues.
Pulse oximetry #
2. Understand the function and limitations of pulse oximetry
Pulse oximetry is an easy, painless, non-invasive method to continuously monitor SpO2. The pulse oximeter is attached to a patient with a finger or ear probe. Beams of red and infrared light pass through the tissue from a transmitter to a receiver. The receiving sensor measures the amount of light absorbed by the oxygenated and deoxygenated Hgb in pulsatile (arterial) blood. They will also monitor a patient’s heart rate by counting the pulsations, and give an indication of signal strength.
Limitations
Motion artifact is the most common cause of inaccuracy with pulse oximeters. Patient movement can cause pulsatile venous flow which will be incorrectly measured as arterial pulsations, causing inaccurate heart rates and oximetry readings.
Poor peripheral perfusion is another common cause of inaccuracy. When using the finger probe, check the proximal pulse and capillary refill closest to the site you plan to use. You may choose to use one hand rather than the other based on your assessment. Look for tight clothes, wrist restraints, tightly taped IV’s, BP cuffs, arterial lines as potential causes of restricted circulation. If the extremities are cold, you may try to warm the person’s hands or increase perfusion by applying heated towels or blankets.
They can be used with patients who breathe through their mouth or nose, as long as the nares are patent. In mouth breathers they may not be as effective, as the oxygen flow will fill the nasopharynx and is then inhaled on inspiration. Nasal cannula are designed to be used with flows of 1 to 6 L/min and have the capacity to deliver FiO2 ranging from 0.24 to 0.44. A guideline to estimate FiO2 is with every 1 L/min increase in oxygen flowrate, the FiO2 can increase by 4%, as shown in the table below.
Limitations #
Check that your patient’s finger is clean, dry, and that the patient does not have false nails or nail polish on. Nail polish should be removed for accurate readings. These factors will influence the light transmission through the finger.
Bright ambient light may affect readings, as well as optical shunting – when light from the transmitter passes directly into the receiver without going through the finger. This may happen if the probe is not positioned properly. If the patient is restless, the probe may be lightly taped onto the finger. Care must be taken that the tape is not tight enough to restrict circulation, as this may alter the SpO2 results.
SpO2 (oxygen saturation measured by pulse oximetry) is not as accurate as SaO2 (oxygen saturation measured by a cooximeter). The pulse-oximeter is designed to distinguish between oxygenated and deoxygenated hemoglobin, but is not able to distinguish between oxygenated hemoglobin and carboxyhemoglobin or methemoglobin. For example, if a patient was exposed to carbon monoxide the pulse oximeter may read SpO2 = 99%. However, if we were to take a blood sample and measure the saturation on a blood gas machine, we may see that the actual arterial saturation SaO2 = 70%, while the carboxyhemoglobin level is 29%. Note that the PaO2 may be normal on this patient, and their skin color is likely still “pink”, but they will not be delivering adequate oxygen to the tissues and will be hypoxic. Methemoglobin can accumulate in patients receiving nitrate-based drugs (nitroglycerine, isordil, cardilate, nitroprusside, nitric oxide) and will also cause falsely high oximetry measurements. The definite test for variant forms of hemoglobin is an arterial blood gas with cooximetry.
Anemic patients with low Hgb may still have normal SpO2, even though O2 available is not enough to meet metabolic demands.
Patients with elevated bilirubin concentrations may have falsely low SpO2 readings.
Cardiac intravenous dyes interfere with light absorption and may cause inaccurate oximetry readings.
Assessment of Accuracy #
Pulse oximeters are a useful tool in assessing oxygenation, but have many limitations. It is important to know how to check for accuracy.
All pulse oximeters have an indicator of signal strength. This may be a bar graph, an audible tone, a waveform, a flashing green/amber/red light, LED lights or some other indicator to show how strong the receiving signal is. If the signal strength is poor, measurements are likely inaccurate.
Most pulse oximeters will also indicate heart rate by counting the number of pulsatile signals. Counting the patient’s heart rate by taking their pulse and comparing it to the heart rate shown on the machine will help you assess the accuracy of the reading.
Pulse oximeters can also have malfunctioning components – the probe, transmitter, cord and the machine itself may be in a state of disrepair. To check the machine, clean the probe and try it on your own finger to assess its function.
Use your patient assessment skills to check the accuracy of a pulse oximeter. If the SpO2 reading does not match the patient’s clinical picture, you may want to confirm accuracy by measuring with a different oximeter or by checking an arterial blood gas for a more definitive SaO2.
Causes of Hypoxemia #
Hazards of Pulse Oximetry
Pulse oximetry is considered a safe procedure. However, tissue injury may occur at the measuring site as a result of probe misuse. Pressure sores or burns from prolonged application (>2 hours) have been reported.
There are three basic causes of hypoxemia. All but the first are common and can be encountered in clinical practice.
1. Low inspired oxygen tension Breathing air at pressures less than atmospheric, such as at high altitudes, can cause hypoxemia. For example, the concentration of oxygen in air is 21%. At 760 mm Hg, the inspired partial pressure of oxygen is 159 mm Hg (760 x .21). This is adequate to meet normal oxygenation demands. At high altitudes, the atmospheric pressure decreases but the concentration of oxygen remains the same. For example, at the top of Mount Everest, at an elevation of 29,029 feet, the barometric pressure is 240 mm Hg. The PO2 is only 43 (240 x 0.21). This would give a hemoglobin saturation of approximately 58%.
Hypoxemia from low inspired oxygen tension may be seen in clinical practice if a person is in an enclosed space without adequate ventilation. The enclosed space may be hazardous if it contains toxic gases or a low concentration of oxygen.
2. Alveolar hypoventilation When a patient hypoventilates, the level of oxygen in the alveoli falls, and the level of carbon dioxide increases. Less oxygen is moved into the pulmonary blood flow, causing arterial hypoxemia.
3. Deadspace and Shunt Ventilation and perfusion do not always match up perfectly between the alveoli and pulmonary capillaries. In some areas of the lung, there is too much perfusion and not enough ventilation, causing a shunt where the blood cannot pick up oxygen and unload carbon dioxide. In other areas, there is too much ventilation and not enough perfusion, causing deadspace where oxygen cannot diffuse into the blood.
Oxygen therapy will correct hypoxemia caused by hypoventilation and moderate shunt or deadspace. Oxygen therapy will also help to decrease cardiopulmonary workload. When hypoxemia is present, the cardiopulmonary response system is activated and the patient must increase their respiratory rate and heart rate to compensate. Supplemental oxygen can help to reduce this high cardiopulmonary demand.
oxygen therapy systems #
4. Describe the capabilities and limitations between low flow and high flow oxygen therapy systems
There is a wide range of equipment available to deliver oxygen in a hospital. Selection of the correct device should depend on an accurate assessment of the clinical objectives of the therapy and knowledge of the capabilities and limitations of the various delivery devices. The equipment used to provide oxygen therapy can be classified into two different groups; the low-flow oxygen system, or variable performance equipment, and the high-flow oxygen system, or fixed-performance equipment. When selecting oxygen therapy equipment, the clinician should ask the following questions:
- What FiO2 does the patient need?
- Is consistency and accuracy of FiO2 required?
- Does the patient need humidity?
- Does the patient have an artificial airway?
- Is tolerance and compliance a problem?
Selection should be done carefully in order to provide adequate oxygenation to prevent hypoxemia, while preventing complications resulting from hyperoxygenation.
Low-Flow Oxygen Systems #
Low-flow oxygen systems provide supplemental oxygen at flows ranging from 0 to 15 L/min. Because the normal minute ventilation of an adult is approximately 40 L/min, low-flow systems do not provide enough gas to meet the patient’s total inspiratory flow demands. The 100% oxygen delivered by the low-flow system directly to the airways is therefore diluted by ambient air on inspiration. How much dilution occurs will depend on the oxygen flowrate, the anatomic reservoir (nasal passage and oropharynx), the mechanical reservoir (space inside the mask), and the patient’s inspiratory pattern including inspiratory flow pattern and minute ventilation. With low-flow oxygen systems, therefore, it is difficult to predict the exact FiO2 the patient will receive.
There are three common low-flow oxygen delivery devices available. These include nasal cannula, the simple mask, and the non-rebreathing mask.
Nasal Cannula #
The nasal cannula, or nasal prongs, are probably one of the most common and widely used simple oxygen delivery devices. They are comfortable and well tolerated. They are made of soft plastic, and consist of two small prongs about ½ an inch long, arising from a supply tube. The prongs sit inside the vestibule of the nose, and the supply tubing is designed to hook behind the patient’s ears.
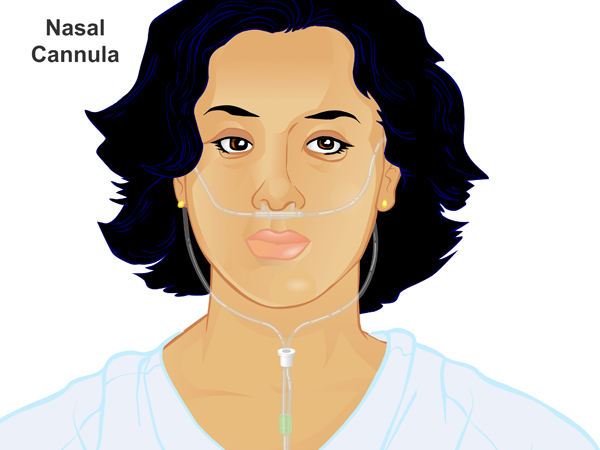
They can be used with patients who breathe through their mouth or nose, as long as the nares are patent. In mouth breathers they may not be as effective, as the oxygen flow will fill the nasopharynx and is then inhaled on inspiration. Nasal cannula are designed to be used with flows of 1 to 6 L/min and have the capacity to deliver FiO2 ranging from 0.24 to 0.44. A guideline to estimate FiO2 is with every 1 L/min increase in oxygen flowrate, the FiO2 can increase by 4%, as shown in the table below.
Approximate FiO2 via nasal cannula
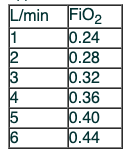
Nasal cannula are generally not used with liter flows greater than 6 L/min, to prevent drying of the nasal mucosa. They are lightweight, economical, disposable, easy to apply, and are very well tolerated by the patient. Complications associated with nasal prongs include instability, as they are easily dislodged by a restless or uncooperative patient, and pressure sores at the crease between the ear and the skull, across the maxilla, and on the nasal septum. They can also cause epistaxis if the nares become too dry, but humidity is generally not required at lower flowrates.
Simple Mask #
The simple face mask is a very common oxygen delivery system for short term use throughout the hospital.
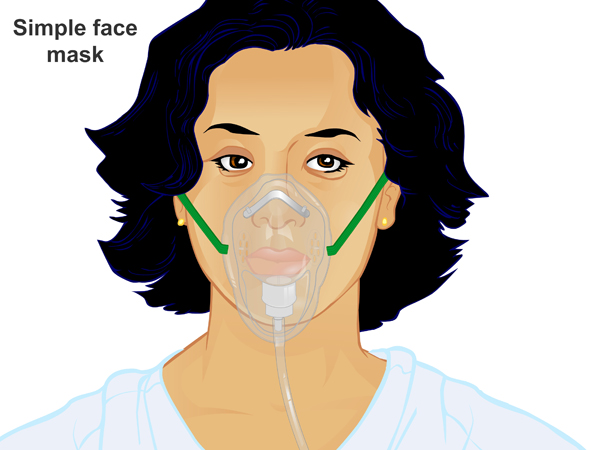
It is made of disposable plastic with holes at the sides for exhalation ports. These holes are also used as an antisuffocation device – if oxygen flow is interrupted, the patient can inhale air through these ports as well as around the edges of the mask. The mask is connected to oxygen supply tubing, and used with flowrates of 6-12 L/min. Care must be taken to ensure the oxygen flowrates are set above 6 L/min to keep the mask clear of carbon dioxide. The simple mask is able to provide FiO2 of approximately 0.40 to 0.60.
There is only one size for adults, which can limit the efficiency of the mask in patients with a poor fit. Because it is a low-flow system, the FiO2 will vary with the patient’s inspiratory flow pattern and minute volume. The FiO2 will also vary depending on the fit of the mask and the amount of air entrainment through the exhalation ports.
Problems associated with the simple mask include an increased potential of aspiration, especially if the mask is tight fitting. It can cause pressure sores on the bridge of the nose and face. A poor fit can cause damage and irritation to the eyes, and some patients may find the mask hot and claustrophobic.
Non-rebreather Mask #
With a non-rebreather mask, there is no rebreathing of exhaled air. It has a series of one-way valves – one between the reservoir bag and the mask, as well as covering both exhalation ports.
On inspiration, the patient inspires from only the reservoir bag; the valves covering the exhalation ports prevent the inspiration of gas from the atmosphere. On exhalation, exhaled gases are prevented from flowing into the reservoir through the valve, and are directed out through the exhalation ports.
The non-rebreather mask is used with oxygen flows of 10 to 15 L/min and sometimes higher to prevent the reservoir bag from collapsing on inspiration. With a good mask fit and high source oxygen flows, FiO2 can range between 0.60 and 0.80, and sometimes approximate 1.00.
With the series of one-way valves in place, these masks have a risk of suffocation if gas flow is interrupted. Patients should never be left alone on a non-rebreather mask for this reason, unless one of the valves covering an exhalation port is removed.
These masks are used by Respiratory Therapists for short term high oxygen delivery such as in-hospital transports or to pre-oxygenate before intubation. They are not available as general ward stock due to the associated risks of suffocation, hyperoxygenation, and lack of humidity.
Recommended flowrates and predicted FiO2 for low-flow systems
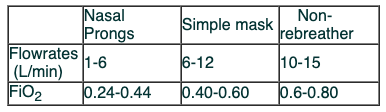
High Flow Oxygen Systems 1 #
High Flow Oxygen Systems
High flow oxygen systems usually consist of a large bottle filled with sterile water, an air/oxygen ratio-ing nebulizer system, a length of corrugated tubing, a drainage bag, and a patient interface.
There are various types of patient interface attachments available for the nebulizers. Corrugated tubing is attached to an aerosol face mask (commonly used), a tracheostomy mask (used to provide oxygen and humidity directly to a tracheostomy), a face tent (used for facial burns, facial injuries, or claustrophobic patients), or a T-piece (attached directly to a tracheostomy or endotracheal tube).
The key factor with any “high flow” oxygen delivery system is that the flowrate from the device exceeds the peak inspiratory flow rate of the patient (normally about 40 L/min and higher with dyspneic patients). There is little additional room air being entrained around the mask, and the FiO2 delivered to the patient’s lungs is close to what we have set on the nebulizer.
High Flow Oxygen Systems 2 #
The nebulizer is connected directly to the oxygen flowmeter, and the flowmeter is set at 15 L/min or higher. The function of the nebulizer is to humidify the inspired gases and to adjust the FiO2. The flow of oxygen that comes from the flowmeter is forced through a tiny jet within the nebulizer that creates a high speed jetstream of oxygen. This jetstream creates a negative pressure that draws water up the straw, and pulls room air in through the entrainment port. The water is broken up into tiny particles (nebulized) for added humidity. The amount of air that is entrained from the room is dependant on the size of the opening on the entrainment port. Turning the dial to a low FiO2 will cause the entrainment port to open further, increasing the amount of air that is entrained from the room. Turning the dial to a higher FiO2 causes the entrainment port to close, decreasing the amount of air entrainment. The total flow that is going to the patient is a combination of the oxygen from the flowmeter, and the amount of air that is entrained.
The higher the FiO2 setting is, the less air is entrained, and the lower the total flow will be going to the patient. At FiO2 settings above 0.40, the FiO2 delivered to the patient may be variable if the patient’s minute ventilation increases, causing air to be entrained from the room. A Respiratory Therapist may need to analyze the FiO2 and add short lengths of corrugated tubing to the aerosol mask, providing a larger oxygen reservoir to prevent FiO2 dilution caused by air entrainment from the room.
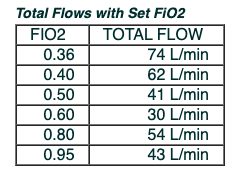
High flow systems on Patient Care Wards #
Nursing staff may be responsible for setting up a high-flow oxygen delivery system when necessary. Review the protocols for your site regarding guidelines for consulting with a Respiratory Therapist.
Algorithm for applying and titrating oxygen therapy #
5. Follow an algorithm for applying and titrating oxygen therapy
Review oxygen therapy titration guidelines at your hospital. See samples below:
Sample Oxygen Titration Guidelines
- SpO2 should be >92%, unless otherwise ordered by a physician.
- Aim to use the lowest FiO2 that will deliver the required SpO2.
- Patient should be at rest, and should not have received bronchodilators in the previous 20 minutes prior to titration.
- Changes in oxygen percentage should be in 5 – 10% increments.
- Changes in liter flow should be in 1 – 2 L/min increments.
- Perform a clinical assessment to determine the effect of oxygen therapy on other body systems (cardio, respiratory, neurologic).
- Perform pulse oximetry (as ordered, or as patient condition warrants from assessment findings) to determine oxygen saturation.
- Recheck the SpO2 and reassess patient’s condition 5 minutes following any change in oxygen delivery.
- Call RT to assess patient when applicable (site-specific).
Titration of Oxygen Algorithm #
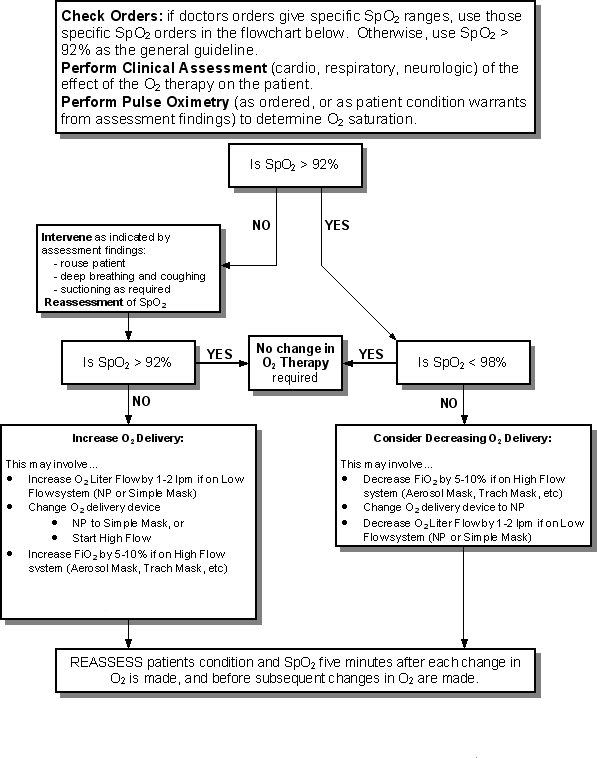
Precautions and complications of oxygen therapy #
6. List precautions and complications of oxygen therapy
Oxygen is both necessary and harmful to life. There are the obvious hazards, such as oxygen supporting combustion, as well as detrimental effects to patients during oxygen administration. Harmful effects depend on the duration of exposure and the PaO2.
Oxygen-Induced Hypoventilation or ‘Hypoxic Drive’
In a person with normal lung function, a stimulation to take another breath occurs when their PaCO2 rises slightly. An increase in PaCO2 stimulates the respiratory center in the brain, creating the impulse to take another breath. In some patients with a chronically high level of PaCO2, such as those with COPD, the stimulus to breathe through this feedback mechanism is blunted and the drive to breathe is caused by a decrease in PaO2. This is called a “hypoxic drive.” In these patients, if given high concentrations of oxygen, their primary drive to breathe is taken away and hypoventilation or apnea may occur. It is important to note that not all COPD patients have chronic retention of CO2, and not all patients with CO2 retention have a hypoxic drive. In fact, it is not often seen in clinical practice.
When administering oxygen to patients with known CO2 retention, watch for signs of hypoventilation, a decreased level of consciousness, and apnea. However, never deprive any patient of oxygen if it is clinically indicated. It is usually acceptable to administer whatever concentration of oxygen the patient needs to maintain the SpO2 between 88 – 92% in patients with known chronic CO2 retention verified by an arterial blood gas.
Absorption Atelectasis
Normally, nitrogen makes up the majority of the gases in the air we breathe and therefore in the alveoli and the dissolved gases in the blood. Breathing 100% oxygen will deplete the level of nitrogen in the alveoli and the blood within 15 minutes. This causes a large drop in the partial pressure of mixed gases in the venous system, called “nitrogen washout.” This will generate a large pressure gradient between the blood and the alveoli. When this happens in the presence of alveoli that are blocked by secretions or debris, the gas in the alveoli will rapidly diffuse into the nitrogen-depleted blood. This causes an increase in the physiologic shunt and resulting hypoxemia.
Oxygen Toxicity #
Oxygen toxicity is a condition that can cause severe damage to the lungs and other organ systems. It is believed to be mediated through chemical units called free radicals. These free radicals in the lung, in the presence of high oxygen concentrations for long periods of time, can inhibit tissue growth, inactivate cellular enzymes, block cellular reproduction, inactivate the regulation of cellular metabolism, and block mitochondrial activity.
The clinical presentation of oxygen toxicity is similar to that of broncho-pulmonary pneumonia, including patchy infiltrates on chest x-ray, alveolar wall edema, and consolidation. These symptoms can lead to hypoxemia and hypoxia.
Although the exact length of time of exposure and partial pressure of oxygen is a subject of debate, it is generally agreed that limiting FiO2 to less than 0.50 if long-term oxygen therapy (greater than 24 hours) is indicated is a good guideline.
Although oxygen toxicity may be severe, lack of oxygen will surely cause tissue and brain damage or death. Oxygen must never be withheld because of potential adverse effects, but it should be carefully titrated to maintain the PaO2 within appropriate levels. Effects of oxygen toxicity have been found to worsen at a FiO2 greater than 0.50. When this level is reached, therefore, try to use other adjuncts to improve oxygenation to avoid toxic levels of oxygen, such as Peep, ventilation, CPAP, physiotherapy, positioning, suctioning, incentive spirometry, bronchodilators, etc. Frequent arterial blood gas levels may be indicated when the patient is on a high level of oxygen in order to titrate to the appropriate PaO2.
Supplemental oxygen should be administered with caution to patients suffering from paraquat (herbicide) poisoning and to patients receiving Bleomycin because these agents have the ability to increase the rate of development of O2 toxicity.
Oxygen Therapy Simulation #
The screen below is an interactive simulation that explores the management of oxygen therapy in the acute clinical setting.
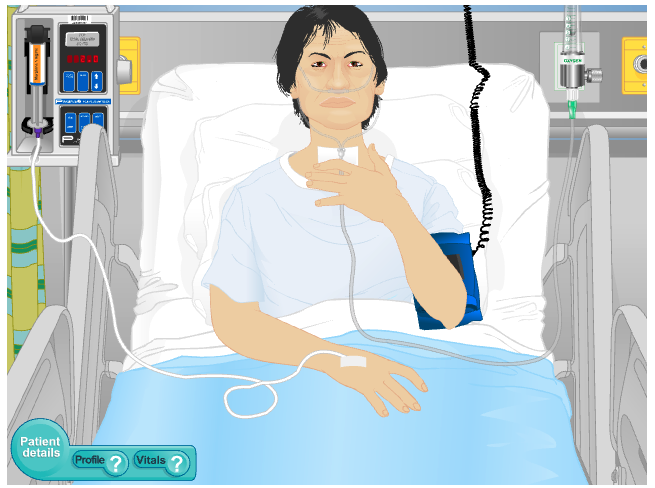
References #
1. Vancouver Acute PCG 0-120 Oxygen Therapy – Initiation and Maintenance
2. Vancovuer Acute PCG 0-130 Oxygen Therapy and Respiratory Care Reference Manual
3. Vancouver Acute PCG D-140 Dyspnea: Care and Management
4. Advanced Ventilation and Respiratory Care – McKeown, S ©2000
5. VGH Pulse Oximetry Module – Bjorndahl, S and Thiessen, R 2004
6. Oxygen Therapy and Pulse Oximetry, Nursing Orientation Module – Thiessen, R 2004
Video: High Flow Setup #
Video: Double Flow Setup #
Video: Oxygen Analyzer Probe #
Video: Pulse Interrupt #
Video: Oxygen Analyzer Probe without ORing #
Cyber Patient contains animated interactive material for e-education.
Cyber Patient Module
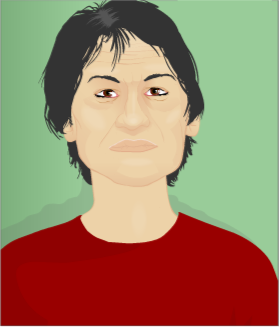
PATIENT PROFILE:
Name: Mrs. Daniel Ngo,
Mrs. Ngo is a 59-year-old female admitted to the surgical ward post-op for total thyroidectomy and radical lymph node resection. Her surgery was complicated by a 2L blood loss. She received 2 units of packed red blood cells and 1L of normal saline bolus during the surgery. She has been having pain in her surgical incision and was assessed by the Post Operative Pain Service (POPS) for pain control. She now has a PCA with Morphine @2mg/dose. L/O time 6 min 4hrs limit 30mg to her left hand IV. She also has IV NS running through her IV at 75 cc/hr.
Current Orders:
- Tylenol 500mg 0 6H prn
- Ativan 1mg sublingual Q4h prn for anxiety
- PCA Morphine @2mg / dose, L / O time 6 min 4hrs limit 30mg
- 0.2mg Hydromorphone Q 2H prn for pain
- Thyroid HRT
- Ambulate as tolerated
- Diet as tolerated